Introduction
Tissue engineering is an interdisciplinary field that combines principles of biology, engineering, and material science to develop functional substitutes for damaged tissues and organs. The primary goal is to restore, maintain, or improve tissue function by leveraging the body’s own regenerative capabilities. This research paper provides a comprehensive overview of tissue engineering, focusing on current trends, techniques, applications, challenges, and future prospects.
Historical Background
The concept of tissue engineering can be traced back to the early 20th century when Alexis Carrel and Charles Lindbergh developed methods to keep tissues alive outside the body. However, it wasn’t until the late 20th century that tissue engineering emerged as a distinct field, driven by advances in stem cell biology, biomaterials, and regenerative medicine.
Core Components of Tissue Engineering
- Cells:
- Stem Cells: Stem cells, particularly mesenchymal stem cells (MSCs) and induced pluripotent stem cells (iPSCs), are pivotal in tissue engineering due to their ability to differentiate into various cell types.
- Primary Cells: Cells harvested directly from tissues, such as chondrocytes for cartilage engineering or hepatocytes for liver tissue, are used in applications requiring specific cell types.
- Scaffolds:
- Natural Biomaterials: Collagen, gelatin, and hyaluronic acid are commonly used natural scaffolds that provide a biocompatible environment for cell growth.
- Synthetic Biomaterials: Polymers such as polylactic acid (PLA) and polycaprolactone (PCL) offer customizable properties and structural support for engineered tissues.
- Hybrid Scaffolds: Combining natural and synthetic materials can enhance the mechanical and biological properties of scaffolds.
- Growth Factors:
- Signaling Molecules: Growth factors like VEGF (vascular endothelial growth factor) and BMP (bone morphogenetic protein) are incorporated into scaffolds to promote cell proliferation, differentiation, and angiogenesis.
- Bioreactors:
- Dynamic Culturing: Bioreactors provide a controlled environment for tissue development, offering mechanical stimulation, nutrient supply, and waste removal, which are crucial for the maturation of engineered tissues.
Techniques in Tissue Engineering
- Scaffold-Based Techniques:
- Electrospinning: This method creates fibrous scaffolds that mimic the extracellular matrix, promoting cell attachment and proliferation.
- 3D Bioprinting: A cutting-edge technique that allows precise deposition of cells and biomaterials to fabricate complex tissue structures layer by layer.
- Cell Sheet Engineering:
- Sheet Harvesting: Cells are cultured to form a cohesive layer and then harvested as intact sheets, which can be layered to form thick, vascularized tissues without the need for scaffolds.
- Decellularization and Recellularization:
- Decellularization: The removal of cells from donor tissues or organs, leaving behind an extracellular matrix scaffold.
- Recellularization: The repopulation of the decellularized matrix with patient-specific cells to create functional tissues.
- Organoids and Microphysiological Systems:
- Organoids: Miniaturized and simplified versions of organs, grown from stem cells, that replicate key aspects of organ structure and function.
- Microphysiological Systems: Also known as “organ-on-a-chip” technologies, these systems use microfluidic devices to model tissue and organ functions in vitro.
Applications of Tissue Engineering
- Skin Tissue Engineering:
- Burn and Wound Healing: Engineered skin grafts, comprising autologous keratinocytes and fibroblasts seeded on biodegradable scaffolds, are used to treat severe burns and chronic wounds.
- Cosmetic Applications: Tissue-engineered skin is also being explored for cosmetic and reconstructive purposes.
- Bone and Cartilage Engineering:
- Orthopedic Implants: Scaffold-based approaches using biocompatible materials and osteogenic cells aim to repair bone defects and enhance the integration of orthopedic implants.
- Cartilage Repair: Engineering cartilage tissues to treat joint defects and osteoarthritis involves the use of chondrocytes or MSCs combined with hydrogels or other supportive scaffolds.
- Cardiovascular Tissue Engineering:
- Heart Patches: Bioengineered cardiac patches, containing cardiomyocytes derived from stem cells, are designed to repair myocardial infarctions.
- Vascular Grafts: Tissue-engineered blood vessels, constructed from endothelial and smooth muscle cells, are developed for bypass surgeries and to replace damaged arteries.
- Nerve Tissue Engineering:
- Peripheral Nerve Regeneration: Techniques involve using conduits filled with supportive cells and growth factors to bridge nerve gaps and promote regeneration.
- Spinal Cord Repair: Scaffold-based approaches combined with neural stem cells aim to restore function in spinal cord injury patients.
- Organ Engineering:
- Liver and Kidney: Research focuses on creating bioartificial organs using decellularized matrices recellularized with hepatic or renal cells to treat liver and kidney failures.
- Lung and Heart: Efforts are underway to engineer functional lung and heart tissues for transplantation and disease modeling.
Challenges in Tissue Engineering
- Vascularization:
- Limitations: One of the primary challenges is ensuring adequate blood supply to the engineered tissues, especially in thick or complex structures.
- Solutions: Approaches include prevascularization of scaffolds, incorporation of angiogenic factors, and co-culturing with endothelial cells to promote vessel formation.
- Immune Response:
- Biocompatibility: Engineered tissues must be biocompatible to avoid adverse immune reactions that can lead to rejection or inflammation.
- Strategies: Using autologous cells, immune-modulatory scaffolds, and gene editing techniques to create hypoimmunogenic cells are potential strategies to overcome immune challenges.
- Scalability and Standardization:
- Manufacturing: Scaling up the production of tissue-engineered constructs while maintaining quality and consistency is a significant hurdle.
- Regulatory Compliance: Developing standardized protocols and meeting regulatory requirements for clinical translation is essential for the widespread adoption of tissue engineering therapies.
- Functional Integration:
- Maturation: Ensuring that engineered tissues mature and integrate functionally with the host tissue remains a critical issue.
- Mechanical Properties: Matching the mechanical properties of engineered tissues with those of native tissues is vital for successful implantation and long-term function.
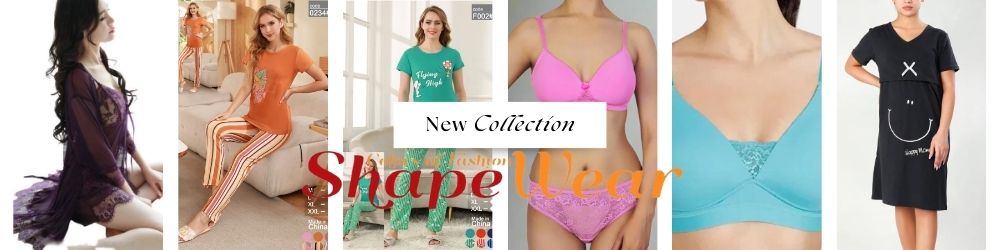
Future Prospects in Tissue Engineering
- Advances in Biomaterials:
- Smart Materials: The development of smart biomaterials that respond to environmental stimuli, such as pH, temperature, or mechanical stress, can enhance the performance and functionality of engineered tissues.
- Biodegradable Polymers: Continued research into biodegradable polymers that can provide temporary support and degrade into non-toxic byproducts is ongoing.
- Stem Cell Innovations:
- Gene Editing: Techniques like CRISPR/Cas9 are being explored to create stem cells with enhanced regenerative capabilities or to correct genetic defects before differentiation.
- Organoids: Advances in organoid technology may provide new insights into organ development, disease mechanisms, and personalized medicine.
- 3D Bioprinting:
- Precision and Complexity: Improvements in 3D bioprinting technology will enable the fabrication of more complex and precise tissue structures, potentially leading to the creation of fully functional organs.
- Bioinks: The development of new bioinks that mimic the extracellular matrix and support cell viability and function is a key area of research.
- Regenerative Medicine and Personalized Therapies:
- Patient-Specific Treatments: Combining tissue engineering with personalized medicine approaches will allow for the creation of customized tissue constructs tailored to individual patients’ needs.
- Regenerative Approaches: Incorporating tissue-engineered constructs into broader regenerative medicine strategies, such as gene therapy and immunomodulation, holds promise for treating a wide range of diseases.
- Clinical Translation and Commercialization:
- Clinical Trials: Ongoing and future clinical trials will provide critical data on the safety and efficacy of tissue-engineered products, paving the way for regulatory approvals and commercialization.
- Industry Collaboration: Collaborations between academic researchers, industry partners, and regulatory agencies will be crucial for advancing tissue engineering from bench to bedside.
Conclusion
Tissue engineering has made significant strides in developing functional tissue substitutes and has the potential to revolutionize regenerative medicine and organ transplantation. While challenges remain, advances in biomaterials, stem cell biology, and bioprinting technology offer promising solutions. The future of tissue engineering lies in personalized therapies, smart biomaterials, and interdisciplinary collaboration, ultimately aiming to improve patient outcomes and address the growing need for tissue and organ replacements.
References
- Langer, R., & Vacanti, J. P. (1993). Tissue engineering. Science, 260(5110), 920-926.
- Murphy, S. V., & Atala, A. (2014). 3D bioprinting of tissues and organs. Nature Biotechnology, 32(8), 773-785.
- Chen, F. M., & Liu, X. (2016). Advancing biomaterials of human origin for tissue engineering. Progress in Polymer Science, 53, 86-168.
- Mao, A. S., & Mooney, D. J. (2015). Regenerative medicine: Current therapies and future directions. Proceedings of the National Academy of Sciences, 112(47), 14452-14459.
- Vacanti, C. A. (2006). History of tissue engineering and a